Key Takeaways:
- The paper describes one process by which chromosomes exposed to the cell cytoplasm break apart.
- The finding sheds light on a key process in cancer and congenital disease
Picture a floor strewn with broken crockery, hastily and haphazardly pieced back together, and you’ll have a sense of the chromosomal chaos inside many cancer cells.
In a phenomenon known as chromothripsis, a chromosome or piece of chromosome shatters and reassembles almost randomly, mangling its genetic information. It’s a common feature of cancer cells, found in 65% of pancreatic cancers, for example, and in virtually all osteosarcomas.
One of the ways chromothripsis can occur is an error during cell division. When a chromosome fails to congregate with its brethren in the center of a new-formed cell, it becomes stranded in its own private nucleus, known as a micronucleus. The membrane, or envelope, of the micronucleus is quite fragile: when it bursts, its resident chromosome, along with a soup of proteins, enzymes, lipids, phosphates and inorganic compounds, spills into the cytoplasm.
The cytoplasm, the gel-like liquid inside the cell, is a surprisingly hostile environment for this kind of castoff chromosome. The chromosome splits into fragments, which scatter throughout the cell. When the cell divides, those fragments randomly wind up in one or the other of the daughter cells. There, the fragments become part of the regular nucleus, where they’re stitched together like the rags in a kite tail, forming a novel, patchwork cancer chromosome.
A forbidding environment
The perils that cytoplasm poses for these chromosomes have been evident for several years. What hasn’t been clear is how this process occurs: what is it about cytoplasm that is so damaging to these straggler chromosomes?
A new study by Dana-Farber scientists provides one answer. Part of the problem, it turns out, has less to do with what’s in the cytoplasm than what tumbles out of the micronucleus.
In the study, researchers led by David Pellman, MD, and Shangming Tang, PhD, generated cells with micronuclei, plucked out the micronuclei, and examined the chromosomal material within them.
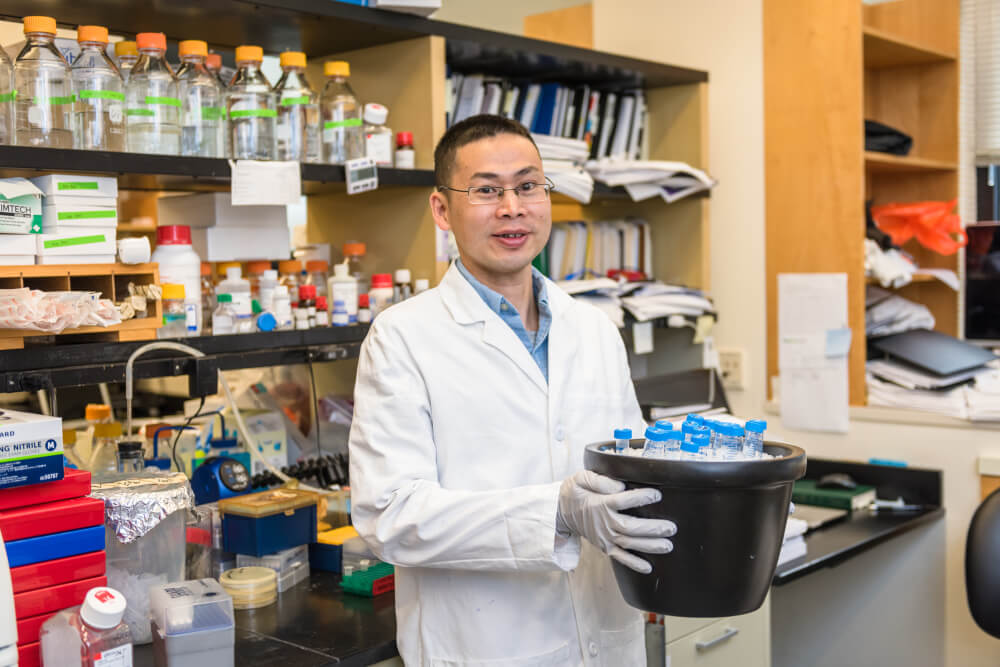
During transcription – the process by which DNA is copied onto RNA to help make proteins – the two strands of the DNA double helix briefly loosen and an RNA molecule binds to one of the strands at a specific location, creating what’s known as a RNA-DNA hybrid. When the Dana-Farber researchers purified the micronuclei from the cells they’d generated, they found a huge throng of RNA-DNA hybrids, far more than is found on chromosomes from a normal cell’s nucleus.
“When the micronuclear envelope breaks, RNA seems to get trapped on DNA, as if it’s caught in the act of transcription,” Pellman remarks.
The abnormal accumulation of RNA-DNA hybrids attracts enzymes that change the letters, or bases, of the genetic code. Other enzymes then try to repair the errors. The repair enzymes swoop in, remove the incorrect base, and make a break or nick in the stand of DNA where the correct base needs to be inserted. In the DNA released from micronuclei, the initial enzymes arrived on the scene and dutifully sliced away at the strands of bases. In a regular nucleus, the sliced DNA would be fixed.
“However, the problem is that when the micronuclear envelope bursts, the full complement of DNA repair enzymes is released into the cytoplasm,” Pellman says. “The DNA-cutting enzymes do their work, but the enzymes involved in sealing the breaks in DNA are lost.”
The researchers found that only chromosomes with large numbers of RNA-DNA hybrids are subject to this kind of mutilation by a thousand cuts. Normal chromosomes, with more modest counts of these hybrids, seem not to be affected.
Pellman notes that this is not the sole process by which micronuclear DNA gets chopped up and indiscriminately reassembled. “Although normal cells rarely have their chromosomes in the cytoplasm, it is common in cancer cells, motivating an intense effort to understand the consequences,” he says.
In addition to chromosome breakage, researchers have found that the presence of DNA in the cytoplasm can trigger an immune response within the cell. The cell behaves as if it has been invaded by viruses and mounts an inflammatory response to rid itself of the unwanted DNA. Many groups are studying whether intensifying this response could augment cancer immunotherapies.
Another consequence, described by Pellman and his colleagues in an upcoming paper, involves changes in the chromatin – or protein packaging – of DNA exiled to the cytoplasm. These changes can alter transcription patterns in ways that may – independent of damage to DNA – promote tumor development or resistance to therapy.
“We’re discovering that the presence of DNA in the cytoplasm can have a variety of consequences for a cell – and, just as importantly, how those consequences occur,” Pellman remarks. “This information is useful not only for understanding a common aspect of cancer but may provide important clues to new treatments for the disease.”